Redefining Cooling: An Expert Analysis of Solar-Driven Adsorption Chillers for Sustainable Data Centers
An Expert Analysis of Solar-Driven Adsorption Chillers for Sustainable Data Centers
I. Executive Summary
The escalating demand for data processing and storage is placing unprecedented strain on energy resources and environmental systems, with data center cooling emerging as a critical area of concern. Conventional cooling technologies contribute significantly to operational expenditures and the overall carbon footprint of these facilities.1 This report provides an in-depth analysis of solar-driven adsorption chiller technology as a viable alternative, examining its scientific foundations, operational characteristics, economic feasibility, and strategic implications for the data center industry.
Solar adsorption cooling, a thermally driven process, offers compelling advantages, particularly in regions with abundant solar resources and water scarcity. Key benefits include substantially reduced electricity consumption for chilling, negligible water use in the core cooling process, and the ability to utilize low-grade waste heat, including return hot water from liquid-cooled IT equipment. Adsorbent materials like silica gels, zeolites, and advanced metal-organic frameworks (MOFs) are central to this technology, operating efficiently under semi-vacuum conditions and driven by thermal energy typically in the range of 55∘C to 90∘C. While the Coefficient of Performance (COP) of adsorption chillers (typically 0.35 to 0.6) is lower than that of conventional electric chillers, the use of solar thermal energy or waste heat as the primary input can lead to significant operational cost savings and environmental benefits.
However, adoption faces challenges, including higher initial capital expenditure (CAPEX) compared to traditional systems, substantial land area requirements for solar thermal collector arrays, and a developing ecosystem for large-scale, mission-critical deployments.2 The technology's readiness for the immense cooling demands of hyperscale data centers requires further validation. The strategic importance of this technology is underscored by converging pressures on data centers: rising energy costs, increasing water scarcity, stringent carbon emission reduction targets, and the strain on electrical grids amplified by the growth of Artificial Intelligence (AI) workloads.3 Adsorption cooling is not merely an energy-saving option but a potential strategic response to these multifaceted challenges.
This report concludes that while solar-driven adsorption chillers present a promising pathway toward more sustainable data center cooling, their widespread adoption, particularly at hyperscale, necessitates focused pilot programs. Such programs are crucial not only for technical validation of performance, reliability, and scalability under real-world operational conditions but also for building the institutional knowledge and operational confidence required by an industry that prioritizes uptime and reliability for its mission-critical infrastructure.
II. The Evolving Landscape of Data Center Cooling
The rapid expansion of digital services, cloud computing, and artificial intelligence has precipitated an exponential increase in the number and capacity of data centers worldwide. This growth brings with it a commensurate rise in energy consumption and environmental impact, with cooling systems representing a major component of this footprint.
A. Escalating Energy Demands and Environmental Imperatives
Global data center energy consumption is substantial and continues to grow. In 2022, data centers and data transmission networks were estimated to account for 1-1.5% of global electricity use, translating to approximately 240-340 TWh.2 Projections indicate that data center energy demands in the U.S. alone could consume as much as 9% of the country's annual electricity generation by 2030.4 Cooling systems are a primary driver of this energy demand, typically accounting for around 40% of a data center's total energy usage 2, and between 60-80% of the non-IT electrical load.1 This significant energy draw contributes to a considerable carbon footprint; data centers were responsible for a 1% share of global Greenhouse Gas (GHG) emissions in 2020, equivalent to 330 Mt CO2.2
The industry is under increasing pressure to align with global Net Zero Emission goals, which necessitate a halving of emissions by 2030.2 Power Usage Effectiveness (PUE), a ratio of total facility energy to IT equipment energy, is a standard industry metric for energy efficiency. While significant strides have been made in reducing PUE values, the sheer growth in IT load means that overall energy consumption continues to rise. Studies on absorption chiller systems, a related thermally driven technology, highlight the potential for emissions reduction when renewable heat sources are employed, even if the PUE might be initially higher than optimized conventional systems.2
The focus on energy efficiency and emissions reduction is no longer solely an operational cost concern but is evolving into a strategic imperative. Environmental regulations are becoming more stringent, and there is growing societal and investor pressure on corporations to demonstrate tangible progress in sustainability. This context elevates the importance of exploring and adopting alternative cooling solutions that can fundamentally reduce reliance on grid electricity and associated emissions.
B. Limitations of Conventional Cooling Technologies
Traditional data center cooling predominantly relies on electrically powered vapor-compression refrigeration systems. These include air-cooled chillers, which expel heat to the ambient air, and water-cooled chillers, which typically use cooling towers that reject heat through water evaporation.1 While effective, these systems have inherent limitations that are becoming increasingly problematic.
Their primary drawback is high electricity consumption. The refrigeration cycle is an energy-intensive process, making chillers, along with their associated fans and pumps, the largest electrical consumers after IT equipment.1 Furthermore, many conventional refrigerants have high Global Warming Potentials (GWPs). Although newer systems are adopting lower GWP refrigerants 6, the legacy impact and potential for leaks from existing systems remain a concern.
Water consumption is another significant issue, particularly for systems employing evaporative cooling towers. A 50MW data center operating at 60% utilization with a PUE of 1.25 and a Water Usage Effectiveness (WUE) of 2.0 can consume approximately 657 million liters (174 million gallons) of water annually.1 In water-scarce regions, this level of consumption is unsustainable and can lead to operational risks and community opposition.3
The increasing power density of IT equipment, especially servers incorporating GPUs for AI and Machine Learning (ML) workloads, further strains conventional cooling. Air-based cooling systems are reported to lose effectiveness when rack densities exceed 20 kW, necessitating a shift towards liquid cooling solutions such as direct-to-chip cooling.3 While liquid cooling can manage higher heat loads more efficiently at the rack level, it still requires a secondary system to reject the captured heat from the facility, often relying on the same conventional chiller technologies.
The limitations of conventional cooling are thus not confined to direct operational costs. They are increasingly becoming strategic liabilities due to environmental regulations, resource constraints (power and water availability), and the social license to operate. The shift towards liquid cooling, driven by high-density AI workloads, presents a unique opportunity. This transition provides higher-grade waste heat in the form of hotter return water from direct-to-chip systems, typically ranging from 55∘C to 70∘C, with some advanced applications reaching 75∘C to 80∘C. These temperatures align well with the activation requirements of thermally driven cooling technologies like adsorption chillers, potentially transforming a cooling challenge into a resource for more sustainable cooling solutions.
III. Solar-Driven Adsorption Chillers: A Technical Deep Dive
Solar-driven adsorption chillers represent a class of thermally powered cooling systems that offer a sustainable alternative to conventional electrically driven chillers. Their operation relies on the physical process of adsorption and desorption of a refrigerant onto a solid adsorbent material, using solar thermal energy or waste heat as the primary energy input.
A. Fundamental Principles of Adsorption Cooling
Adsorption cooling is a thermally driven refrigeration process that utilizes the interaction between a solid adsorbent material and a refrigerant vapor (adsorbate). The basic principle involves the adsorbent material's ability to attract and hold molecules of the refrigerant on its surface at lower temperatures and pressures, and release them when heated. A typical adsorption chiller operates in a cycle involving four key stages: adsorption, desorption, condensation, and evaporation, often occurring within chambers operating under vacuum conditions to facilitate refrigerant evaporation at low temperatures.7
1. Adsorption: The adsorbent material in one bed, cooled from a previous regeneration phase, adsorbs refrigerant vapor from an evaporator. This adsorption process maintains a low pressure in the evaporator, causing the liquid refrigerant (typically water) within it to boil at a low temperature (e.g., 5∘C to 15∘C). This evaporation extracts heat from a chilled water loop, producing the desired cooling effect.
2. Desorption (Regeneration): Simultaneously, in another adsorbent bed saturated with refrigerant from a previous adsorption phase, heat is applied (e.g., from solar thermal collectors or waste heat, typically 55∘C to 90∘C or higher). This thermal energy causes the adsorbent to release the captured refrigerant vapor at a higher pressure.
3. Condensation: The desorbed high-pressure refrigerant vapor flows to a condenser, where it rejects heat to a cooling water loop (e.g., from a dry cooler or cooling tower) and condenses back into a liquid.
4. Evaporation: The liquid refrigerant then passes through an expansion valve, reducing its pressure and temperature before it enters the evaporator, where the cycle repeats.
Most commercial adsorption chillers use two or more adsorbent beds to provide continuous cooling, with one set of beds adsorbing while the other is desorbing. The process is distinct from electrically driven vapor compression cycles as the primary energy input is thermal, significantly reducing electrical consumption. Key components include the adsorbent beds, evaporator, condenser, expansion valve, and the heat source (e.g., solar thermal collectors). The use of water as a refrigerant makes these systems environmentally benign ("green" refrigerant).7
B. Advances in Adsorbent Materials (MOFs, Silica Gels, Zeolites)
The performance of adsorption chillers is critically dependent on the properties of the adsorbent material. Research and development efforts have focused on materials like silica gel, zeolites, activated carbons, and more recently, Metal-Organic Frameworks (MOFs).8
- Silica Gel: This is a widely used adsorbent, often paired with water as the refrigerant. It is known for its relatively low cost, good mechanical properties, and effectiveness in moisture adsorption.8 Commercial systems using silica gel-water pairs are available, with manufacturers claiming low maintenance and long lifespans.7 Experimental studies with silica gel-water systems have reported COPs around 0.37 to 0.403 under specific test conditions.10
- Zeolites: These are crystalline aluminosilicates with well-defined porous structures. They exhibit good thermal stability, making them suitable for temperature swing adsorption processes.8 Like silica gel, they are often used with water as the refrigerant.
- Metal-Organic Frameworks (MOFs): MOFs are a newer class of porous crystalline materials composed of metal ions or clusters coordinated to organic ligands. They offer exceptionally high surface areas and tunable pore structures, leading to potentially higher adsorption capacities compared to traditional materials.9 Some studies suggest MOFs can have an adsorption capacity up to 1.58 times greater than silica gel or zeolites.8 Computational screenings have identified MOFs with potential deliverable working capacities for methanol or ethanol of around 0.6 mL of working fluid per 1 mL of structure, exceeding the highest experimentally measured capacities for MOFs with methanol (ca. 0.45 mL mL−1).9
C. Durability and Stability
Long-term material stability, ideally 10–20 operational years, is a crucial consideration for data center applications.
- Silica gel and zeolites generally offer good stability and mechanical properties.8 Bry-Air, a manufacturer of silica gel-based adsorption chillers, claims a life expectancy of over 25 years for their systems.7
- MOFs, while promising in capacity, can present stability challenges. Several MOFs have been shown to lose structural integrity after prolonged exposure to water, which is problematic as water is an otherwise ideal and environmentally friendly refrigerant.9 This degradation can be less severe or absent when alcohols like methanol or ethanol are used as working fluids with certain MOFs, but these alternatives introduce other considerations such as flammability or toxicity.9 The flexibility of some MOF structures can also lead to desorption hysteresis, which is thermodynamically unfavorable and can reduce system efficiency.9
- Advancements in composite materials, such as incorporating activated carbon with polymers or inorganic substances, aim to enhance thermal conductivity and mechanical strength, which are vital for long-term durability.8 The development of "smart" adsorbents responsive to external stimuli and nanoscale pore engineering are also active research areas.8
The choice of adsorbent material involves a trade-off between performance (capacity, kinetics), stability, and cost. While advanced materials like MOFs may offer higher COPs or Specific Cooling Powers (SCPs), potentially reducing the physical size of the chiller or the required solar collector area for a given cooling capacity, their current cost and demonstrated long-term stability, particularly with water, require careful evaluation. A higher initial investment in a more efficient but potentially less durable or more expensive MOF might not yield a better Total Cost of Ownership (TCO) if replacement cycles are shorter or operational risks are higher. This necessitates thorough techno-economic analysis early in the system design phase, considering the entire lifecycle.
The following table provides a comparative overview of key adsorbent materials:
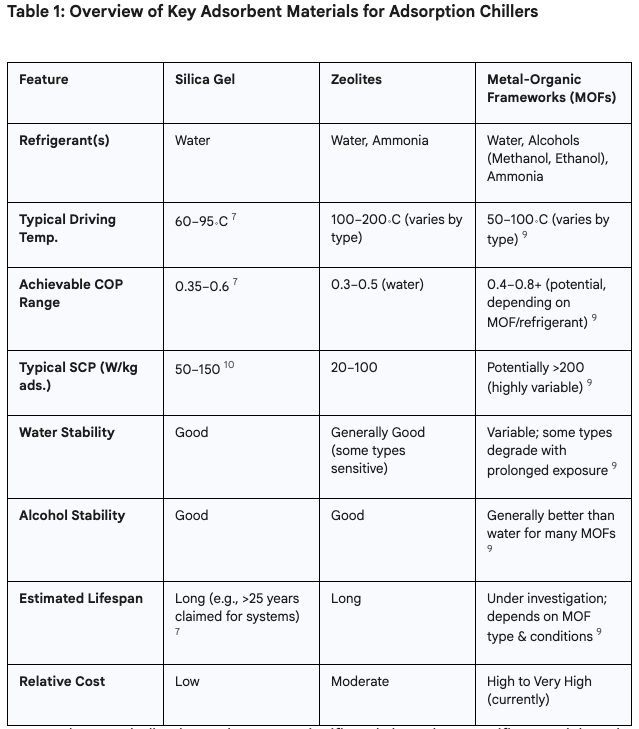
Note: Values are indicative and can vary significantly based on specific material grades, operating conditions, and system design.
D. Key Operational Parameters and Performance Metrics
Understanding the operational parameters and performance metrics is essential for evaluating the feasibility and effectiveness of adsorption chillers.
- Vacuum Conditions: Adsorption chillers operate under vacuum to enable the refrigerant (typically water) to evaporate at low temperatures suitable for cooling. Typical operating pressures within the evaporator and adsorber are in the semi-vacuum range of approximately 50–400 mbar (5,000–40,000 Pa). Optimal cycles are often observed near 100–150 mbar (10,000–15,000 Pa). Higher-performance systems, potentially using advanced adsorbents or specific cycle designs, might achieve deeper vacuum levels approaching 10 mbar (1,000 Pa) to enable even lower desorption temperatures or faster cycle regeneration.
Temperatures:
- Driving Heat Source Temperature: This is the temperature of the hot water or steam supplied to the desorber to regenerate the adsorbent. Adsorption chillers are valued for their ability to utilize low-grade heat. Activation temperatures often range from 55∘C to 90∘C. Solar thermal collectors can achieve temperatures up to 120−180∘C, providing ample driving heat. Commercial systems like those from Bry-Air specify optimal hot water inlet temperatures around 90∘C 7, while research systems have been tested with 80∘C hot water.10
- Chilled Water Output Temperature: This is the temperature of the water produced by the chiller for cooling purposes. Commercial systems can provide chilled water between 5∘C and 10∘C.7 For instance, specific models from Bry-Air list a chilled water outlet temperature of 7.2∘C with an inlet of 12.8∘C.7
- Cooling Water (Condenser) Temperature: This is the temperature of the water used to remove heat from the condenser. Typical inlet temperatures for cooling water range from 24∘C to 35∘C.7 Test conditions in research have used 30∘C cooling water.10 The efficiency of the chiller is sensitive to this temperature; lower cooling water temperatures generally improve performance.
- Coefficient of Performance (COP): The COP for cooling is defined as the ratio of the cooling output (heat removed by the evaporator) to the heat input supplied to the desorber. For adsorption chillers, COP values are generally lower than those of conventional electric chillers.
- Silica gel-water systems have demonstrated experimental COPs of 0.37 and average field test COPs of 0.403.10
- Commercial silica gel-based adsorption chillers claim COPs around 0.55.7
- It is important to distinguish these from absorption chillers, which are also thermally driven but operate on a different principle (absorption into a liquid solution). Absorption chillers can have COPs ranging from 0.6 to 1.4, depending on the cycle (single-effect, double-effect).11 The primary advantage of adsorption chillers is not necessarily a high COP, but their ability to use low-cost or "free" thermal energy (solar, waste heat), thereby reducing reliance on expensive electricity.2 The practical COP achieved in a data center environment is not a static figure but will fluctuate based on the available heat source temperature (which can vary with solar conditions or IT load if waste heat is used), the required chilled water setpoint, and ambient conditions influencing the condenser water temperature. This dynamic nature makes performance modeling more complex than for electric chillers, where COP is more directly related to load and ambient temperature.
- Specific Cooling Power (SCP): SCP is defined as the cooling power generated per unit mass of adsorbent material (typically in W/kg). It is an indicator of the compactness of the adsorbent bed. A study reported an SCP of about 72 W/(kg adsorbent) for a silica gel-water system.10 Improving SCP is a key research goal, as it can lead to smaller, more cost-effective chiller designs.8
E. Integration with Solar Thermal Systems and Waste Heat Sources
A key advantage of adsorption chillers is their ability to be driven by various low-temperature heat sources.
-Solar Thermal Collectors: These are the most commonly discussed renewable heat source. Various types of collectors can be used:
- Flat plate collectors are suitable for lower temperature requirements (typically up to 80−90∘C).
- Evacuated tube collectors can achieve higher temperatures (up to 120−150∘C) more efficiently, making them well-suited for driving adsorption chillers.
- Concentrating solar collectors (e.g., parabolic troughs) can achieve even higher temperatures (150−400∘C), which could be used for advanced adsorption cycles or other thermal processes, though they are more complex and require direct sunlight. The ability to harness solar thermal energy at temperatures up to 120−180∘C effectively matches the needs of many adsorption chiller designs.
- Waste Heat Sources: Low-grade industrial waste heat or waste heat from electronic equipment, particularly from data centers themselves, can be an excellent energy source. The trend towards liquid cooling in data centers makes this synergy particularly strong, as the return water from direct-to-chip cooling systems is often in the ideal temperature range for adsorption chiller activation.12
- Phase Change Materials (PCMs): PCMs can be integrated for thermal energy storage. This allows for the storage of solar thermal energy collected during peak sunshine hours for later use, or for buffering intermittent waste heat supply. This capability enhances system reliability and can enable cooling during periods when the primary heat source is unavailable, such as at night or during cloudy weather for solar-driven systems.
The integration of these heat sources, particularly waste heat from the data center itself, can create a virtuous cycle, reducing both cooling energy demand and the load on heat rejection systems.
IV. Strategic Convergence: Adsorption Cooling and Modern Data Center Architectures
The rise of high-density computing and increasing focus on sustainability are reshaping data center design. Solar-driven adsorption chillers offer several strategic advantages that align well with these evolving architectures, particularly in conjunction with liquid cooling and the imperative for enhanced resource efficiency.
A. Synergies with Direct-to-Chip Liquid Cooling
Direct-to-chip liquid cooling is becoming increasingly prevalent as a solution to manage the intense heat generated by high-performance processors and GPUs, especially in AI/ML clusters.3 This cooling method captures heat more effectively at the source, resulting in significantly higher coolant return temperatures compared to traditional air cooling. Typical return water temperatures from direct-to-chip systems range from 55∘C to 70∘C, with advanced applications pushing this to 75∘C or even 80∘C.
These temperatures are a crucial asset when considering adsorption chillers. Many adsorption chiller designs are optimized for activation with hot water in the 55∘C to 90∘C range.7 Some systems can effectively utilize heat as low as 50∘C.7 This thermal alignment means that the "waste" heat from liquid-cooled IT equipment, which would otherwise need to be rejected to the atmosphere, can be directly repurposed as the primary energy input for an adsorption chiller.12 This synergy transforms a thermal management liability into a valuable resource, directly fueling the cooling process and reducing the need for external heat sources like dedicated solar thermal arrays or boilers.
This integration can simplify the overall thermal management architecture. If the return water from liquid cooling loops is already at a sufficiently high temperature, it may directly feed the adsorption chiller's generator. This could potentially eliminate the need for intermediate heat exchange loops or heat pumps that would otherwise be required to boost lower-grade waste heat to a usable temperature, as is sometimes considered for district heating applications.12 Such simplification could reduce system complexity, capital costs associated with waste heat recovery, and parasitic energy losses.
B. Enhanced Water Efficiency and Potential for Water Reclamation
Water consumption is a major operational and environmental concern for data centers, especially those relying on evaporative cooling towers.1 Adsorption chillers offer a compelling solution to this challenge.
The core adsorption cooling process typically uses water as a refrigerant in a closed loop. This means that, unlike cooling towers, the chiller itself does not consume water through evaporation for heat rejection from the primary cooling cycle. The only water involved is the refrigerant itself, which is continuously cycled within the sealed system. This results in negligible process water consumption for the chilling effect. Furthermore, during the adsorption cycle, particularly when processing return air with some humidity in an air conditioning application, the chiller can condense airborne moisture. This condensate is often pure distilled water and can be collected. Estimates suggest a potential to produce 0.1–0.3 liters of condensate per kWh of cooling provided. In arid or semi-arid climates, where many data centers are being built due to land availability or renewable energy access, this reclaimed water can be a valuable supplementary source for other facility needs (e.g., humidification, greywater systems) or can contribute to achieving water-positive operational goals.
This contrasts sharply with the substantial water footprint of conventional cooling towers. For example, a 50MW data center with a PUE of 1.25 and a WUE of 2.0 (liters of water per kWh of IT energy) might consume 657 million liters of water annually.1 By significantly reducing or eliminating this evaporative water loss, adsorption chillers can drastically improve a facility's overall water usage effectiveness. This water efficiency benefit is particularly amplified in regions that experience both high solar irradiance (favorable for solar thermal input) and significant water stress – conditions that often coexist in areas like the US Southwest, the Middle East and North Africa (MENA) region, and parts of Southern Europe. For data centers in these geographies, the dual benefit of solar energy utilization and water conservation makes adsorption cooling a highly strategic option.
C. Energy Cost Reduction and Peak Load Shaving Capabilities
By utilizing solar thermal energy or readily available waste heat, adsorption chillers can significantly reduce the electrical energy consumed by conventional chiller plants. This directly translates to lower electricity operational expenditures (OPEX). While absorption chillers are a different technology, studies indicating 50-90% electricity use reduction after their installation (when waste heat is utilized) point to the potential savings achievable by thermally driven cooling systems.11 The primary electrical load for an adsorption chiller system comes from pumps for circulating hot water, cooling water, and chilled water, as well as control systems and fans for heat rejection if dry coolers are used. These parasitic loads are typically much lower than the compressor loads of electric chillers.
The integration of thermal energy storage (TES) systems, such as hot water tanks or Phase Change Materials (PCMs), further enhances the economic and operational benefits. TES allows solar energy collected during peak daylight hours to be stored and used to power the adsorption chiller during periods with no or low solar radiation, or during times of peak electricity grid pricing. This capability enables peak load shaving, reducing demand charges which can form a significant portion of a data center's electricity bill. Underground Thermal Energy Storage (UTES) is another concept being explored for storing "cold" energy, which can be discharged during peak grid load hours, effectively acting like a thermal battery.4 While UTES is different from hot thermal storage for adsorption chillers, the principle of using thermal storage to decouple cooling generation from peak electrical demand is similar and strategically valuable for reducing OPEX and improving grid stability.
V. Economic Feasibility and Total Cost of Ownership (TCO)
The economic viability of solar-driven adsorption chillers in data centers hinges on a comprehensive analysis of their Total Cost of Ownership (TCO) compared to conventional cooling technologies. This involves evaluating Capital Expenditures (CAPEX), Operational Expenditures (OPEX), and other financial factors over the system's lifespan.
A. Comparative Analysis: CAPEX, OPEX, and TCO vs. Conventional Cooling
-
Capital Expenditures (CAPEX): Adsorption chiller systems generally entail higher upfront CAPEX compared to conventional electric chiller systems. Studies on thermally driven absorption chillers (often used as a proxy for initial cost comparisons due to their thermal drive nature) suggest their system cost can be at least four times higher than that of a free-cooling electrical chiller system.2 One source indicates an average CAPEX for absorption chillers around $600/kW-th (thermal kilowatt of cooling capacity).11
The major CAPEX components for a solar adsorption cooling system include:
- The adsorption chiller unit(s).
- The solar thermal collector array (e.g., evacuated tubes, flat plates), including mounting structures and land.
- Thermal energy storage (hot water tanks, PCMs).
- Pumps, piping, valves, and control systems.
- Heat rejection equipment for the condenser (e.g., dry coolers or, if existing, cooling towers). In contrast, conventional system CAPEX typically includes 1:
- Electric chiller units.
- Cooling towers and associated water treatment systems (for water-cooled chillers) or air-cooled condensers/dry coolers.
- Pumps, piping, and controls. The initial cost of chillers can be estimated using methods like the six-tenth rule, which scales costs based on capacity relative to a reference unit, but specific reference data for large-scale adsorption chillers is less readily available.13
- Operational Expenditures (OPEX):
- This is where adsorption chillers aim to provide significant advantages.
- Adsorption System OPEX: The primary energy input, solar thermal energy, is effectively free after the initial investment in collectors. If waste heat is used, its cost is also typically very low or zero. The main electricity consumption comes from parasitic loads (pumps, controls, fans for dry coolers), which are considerably less than compressor loads in electric chillers. Maintenance costs are projected to be lower due to fewer moving parts in the adsorbent beds (no large compressors) and the use of water as a refrigerant, which avoids issues related to synthetic refrigerant handling and potential leaks.7 Bry-Air claims low maintenance for their adsorption chillers.7
- Conventional System OPEX: Dominated by electricity costs for chillers, fans, and pumps. Cooling systems can account for 60-80% of a data center's non-IT electricity consumption.1 For chilled water plants (CWPs), OPEX typically constitutes 70-85% of the TCO, and can even reach 90% in some cases.13 Maintenance of compressors, fans, and regular water treatment for cooling towers also contribute significantly to OPEX. General data center OPEX breakdowns show maintenance as a large component (c40%) and electricity as 15-25% of total facility OPEX.14
-
Total Cost of Ownership (TCO):
The economic case for solar adsorption chillers relies on the premise that substantially lower OPEX (primarily from "free" thermal energy and reduced maintenance) will offset the higher initial CAPEX over the system's operational lifetime (e.g., 15-25 years).
The viability is highly sensitive to the actual achievable solar fraction (the percentage of the total cooling load that can be met by solar thermal energy) and the cost and efficiency of any backup thermal source. If, for instance, a significant portion of the thermal energy for desorption must be provided by electric heating due to insufficient solar availability or waste heat, the PUE and OPEX benefits diminish rapidly. An absorption chiller system operated primarily by electricity for its heat source can have a PUE above 2.0, far worse than a typical free-cooling electric chiller system's PUE of below 1.3.2
Furthermore, TCO calculations must meticulously account for the lifespan and potential degradation or replacement costs of both the solar thermal field and the adsorbent material itself. While claims of "minimal maintenance" for the core adsorption beds may be valid 7, the entire system, including solar collectors, pumps, and controls, will require regular upkeep. Concerns about the long-term stability of some advanced adsorbent materials, like certain MOFs when exposed to water 9, could lead to periodic replacement costs, impacting TCO. If adsorbents or critical components require replacement more frequently than anticipated (e.g., every 5-10 years instead of a projected 15-20 years), the lifecycle cost benefits could be significantly eroded.
The following table provides an indicative structure for comparing TCO components:
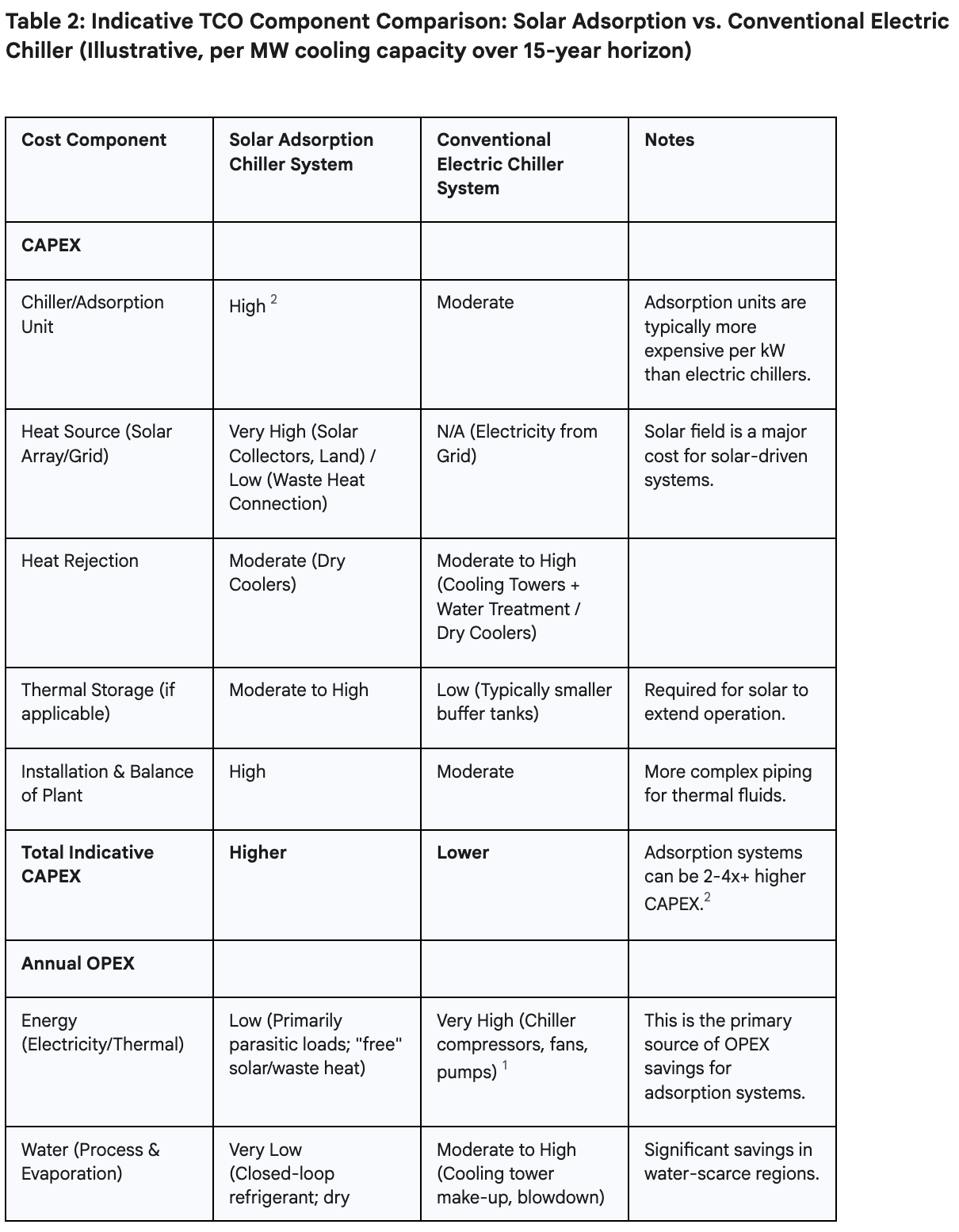
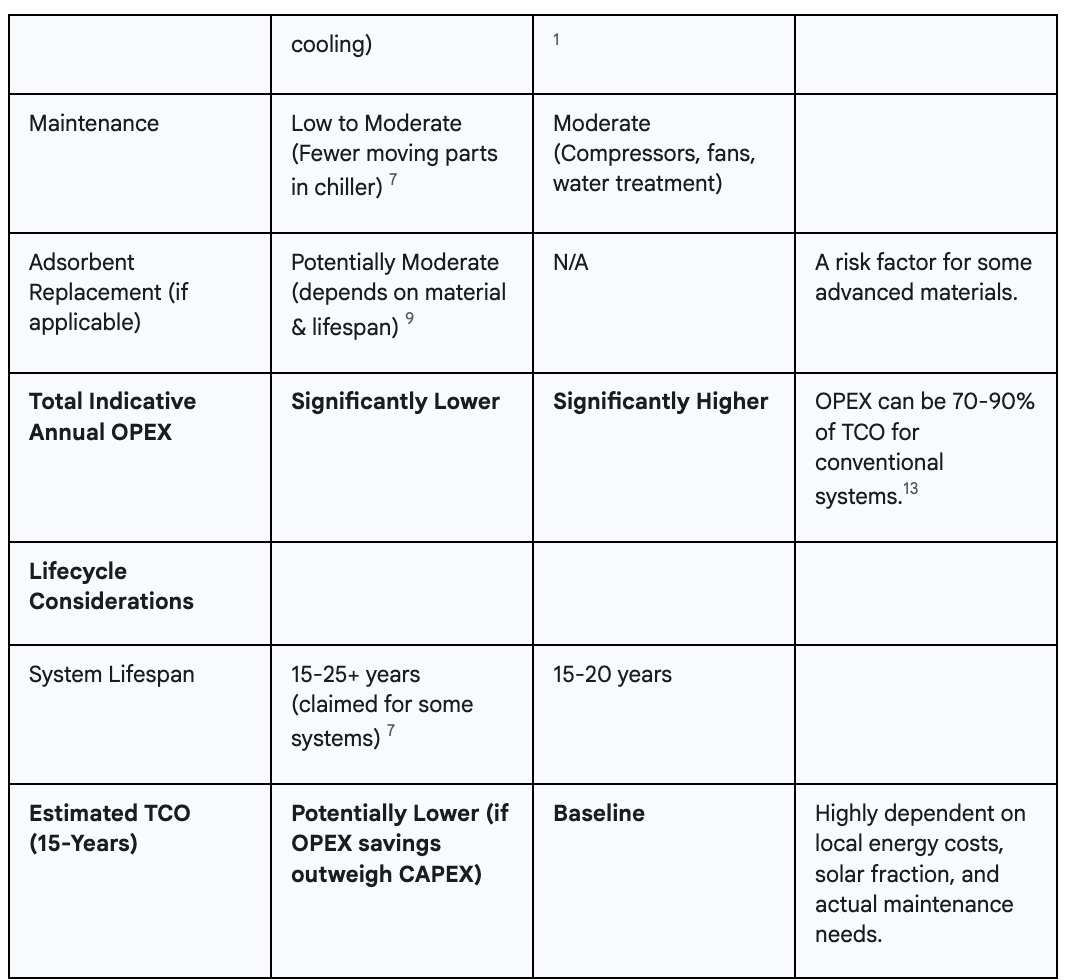
Note: This table is illustrative. Actual costs vary widely based on project specifics, location, scale, and technology choices.
B. Factors Influencing Levelized Cost of Cooling (LCOC)
The Levelized Cost of Cooling (LCOC) is a metric that annualizes the total lifecycle cost of a cooling system and divides it by the annual cooling energy delivered, providing a cost per unit of cooling (e.g., $/kWh-cooling or $/ton-hour). It allows for a more equitable comparison of different cooling technologies with varying CAPEX, OPEX, and lifespans.15
Key factors influencing the LCOC for a solar adsorption cooling system include:
- Initial CAPEX: Higher CAPEX directly increases LCOC.
- Cost of Capital: Interest rates on loans for the initial investment.
- System Lifetime: Longer operational life spreads the CAPEX over more years, reducing LCOC. This includes the lifespan of solar collectors, the adsorption chiller unit, and particularly the adsorbent material.
- Annual Cooling Demand & Utilization: Higher utilization of the cooling system reduces LCOC as fixed costs are spread over more cooling output.
- Price of Electricity: Affects parasitic loads for adsorption systems and is the primary energy cost for conventional systems. Higher electricity prices make adsorption systems more competitive.
- Price of Thermal Energy: If solar or waste heat is not entirely "free" (e.g., opportunity costs, maintenance of waste heat recovery systems), this adds to OPEX.
- Operations and Maintenance (O&M) Costs: Includes routine maintenance, repairs, and potential replacement of components like adsorbent materials.
- Solar Collector Efficiency & Degradation: Affects the amount of "free" thermal energy available over the system's life.
- Adsorption Chiller COP: Higher COP means less thermal input (and thus smaller solar field) for the same cooling output, reducing both CAPEX and LCOC.
- Government Incentives/Subsidies: Tax credits or rebates for renewable energy systems can reduce effective CAPEX and LCOC.
A study on a solar absorption system (related technology) coupled with thermal storage for a shopping center indicated LCOC values of 0.034/kWh with chilled water storage and 0.049/kWh without, demonstrating the potential for cost reductions through optimized system design and thermal storage strategies.16
C. Impact of Carbon Pricing and Sustainability Mandates
The broader economic context, including environmental regulations and corporate sustainability initiatives, can significantly influence the financial attractiveness of solar adsorption cooling.
- Carbon Pricing: The implementation of carbon taxes or emissions trading schemes (ETS) adds a direct cost to greenhouse gas emissions. Since solar adsorption cooling, when driven by solar or true waste heat, has a very low operational carbon footprint compared to conventional systems reliant on grid electricity (especially in regions with carbon-intensive grids), carbon pricing improves its relative economic viability.
- Sustainability Mandates & ESG Goals: Many corporations have established ambitious sustainability goals, including achieving net-zero emissions, carbon neutrality, or water positivity. Investing in technologies like solar adsorption cooling can help meet these targets. While these systems might have higher upfront costs, the long-term environmental benefits and alignment with corporate Environmental, Social, and Governance (ESG) criteria can justify the investment beyond a simple payback calculation. Data centers that demonstrate superior sustainability and resource efficiency may also gain a competitive advantage in attracting environmentally conscious clients and in securing permits and social license to operate, particularly in resource-constrained or environmentally sensitive regions.
VI. Deployment Considerations for Solar Adsorption Chiller Systems
While solar-driven adsorption chillers offer compelling benefits, their practical deployment in data centers, especially at hyperscale, involves several critical considerations ranging from geographic suitability and land requirements to technology readiness and vendor support.
A. Optimal Geographic Regions and Climatic Suitability
The performance and economic viability of solar-driven adsorption cooling systems are intrinsically linked to local climatic conditions. Ideal regions are characterized by:
- High Direct Normal Irradiance (DNI): Abundant solar radiation is essential for solar thermal collectors to efficiently generate the required hot water temperatures. Regions with DNI levels exceeding 5.5–7.5 kWh/m²/day are particularly favorable.
- Low-to-Moderate Relative Humidity: While not directly impacting the chiller's internal operation (which uses a closed refrigerant loop), ambient humidity can affect the performance of dry coolers used for heat rejection from the condenser. More importantly, high ambient temperatures, often correlated with high solar radiation, drive up cooling demand, making efficient cooling solutions more valuable.
- Water Scarcity: In arid or semi-arid climates where water resources are constrained, the negligible water consumption of adsorption chillers (when paired with dry coolers for heat rejection) becomes a significant advantage over systems using evaporative cooling towers.
Based on these factors, the user's article correctly identifies suitable geographies, including:
- The US Southwest (e.g., Arizona, West and South Texas)
- Southern Europe (e.g., Spain, Italy, Greece)
- The North African corridor (e.g., Morocco, Egypt, Tunisia)
- Middle Eastern Gulf States (e.g., UAE, Saudi Arabia, Jordan)
A study analyzing the feasibility of solar-assisted absorption chillers (a related thermally driven technology) across five global locations found Kuwait City to be the most favorable.2 This was attributed to its high year-round solar irradiation and a fossil-fuel-dominant electricity mix, which means that displacing electric chillers with solar thermal systems yields substantial carbon emission reductions.2 These regions often face pressures to reduce water consumption and diversify energy sources, aligning well with the strengths of solar adsorption cooling.
B. Land Footprint Analysis: Solar Collector Area and System Space Requirements
A significant challenge for solar-driven adsorption cooling is the physical space required, both for the chiller plant itself and, more critically, for the solar thermal collector array.
- Chiller Plant Area: Adsorption chiller systems, including the chiller units, thermal storage tanks, pumps, and piping, may require 1.5 to 2.5 times more indoor or covered floor area per ton of cooling compared to conventional electric chiller plants. This is due to the generally lower energy density of thermal processes and the need for larger heat exchangers and adsorbent beds. However, this can be partially offset by savings in space otherwise allocated to large cooling towers and their associated infrastructure.
- Solar Collector Area: The land required for the solar thermal collector array is often the most substantial spatial constraint.
- Estimates for photovoltaic (PV) solar arrays, which convert sunlight to electricity, range from 3 to 5 acres per MW of electrical output.17 Powering a 100 MW data center entirely with PV, accounting for capacity factors and storage, could hypothetically require over 1,400 acres.18
- Solar thermal collectors are generally more efficient at converting solar radiation into heat than PV cells are at converting it into electricity. Global data on large-scale solar thermal systems (for various applications, not just cooling) indicates an average of approximately 1,420 m² of collector area per MW of thermal capacity (MWth).19 This translates to roughly 0.142 hectares/MWth or 0.35 acres/MWth.
- To estimate the area for data center cooling, one must consider the adsorption chiller's COP. If a chiller has a COP of 0.5, it requires 2 MWth of heat input to produce 1 MW of cooling (MWc). Thus, for 1 MWc, 2 MWth×0.35 acres/MWth=0.7 acres of solar collectors would be needed, assuming the collectors operate at their rated thermal output. Actual area will depend on specific collector efficiency, solar irradiance, and desired solar fraction.
This land requirement can be a major barrier, especially for data centers in urban or land-constrained areas, or for retrofitting existing facilities. While innovative concepts like integrating solar thermal collectors into building facades, rooftops, or even pavements are being explored 20, their applicability to meet the concentrated multi-megawatt cooling demands of large data centers is yet to be proven at scale. For a 10 MW cooling load, even with efficient collectors and a good COP, the solar field could easily occupy 5-10 acres or more. This constraint makes solar adsorption cooling more feasible for new-build data centers in locations with ample, affordable land, or facilities co-located with industrial plants that can provide substantial waste heat.
The following table illustrates estimated solar thermal collector area requirements:
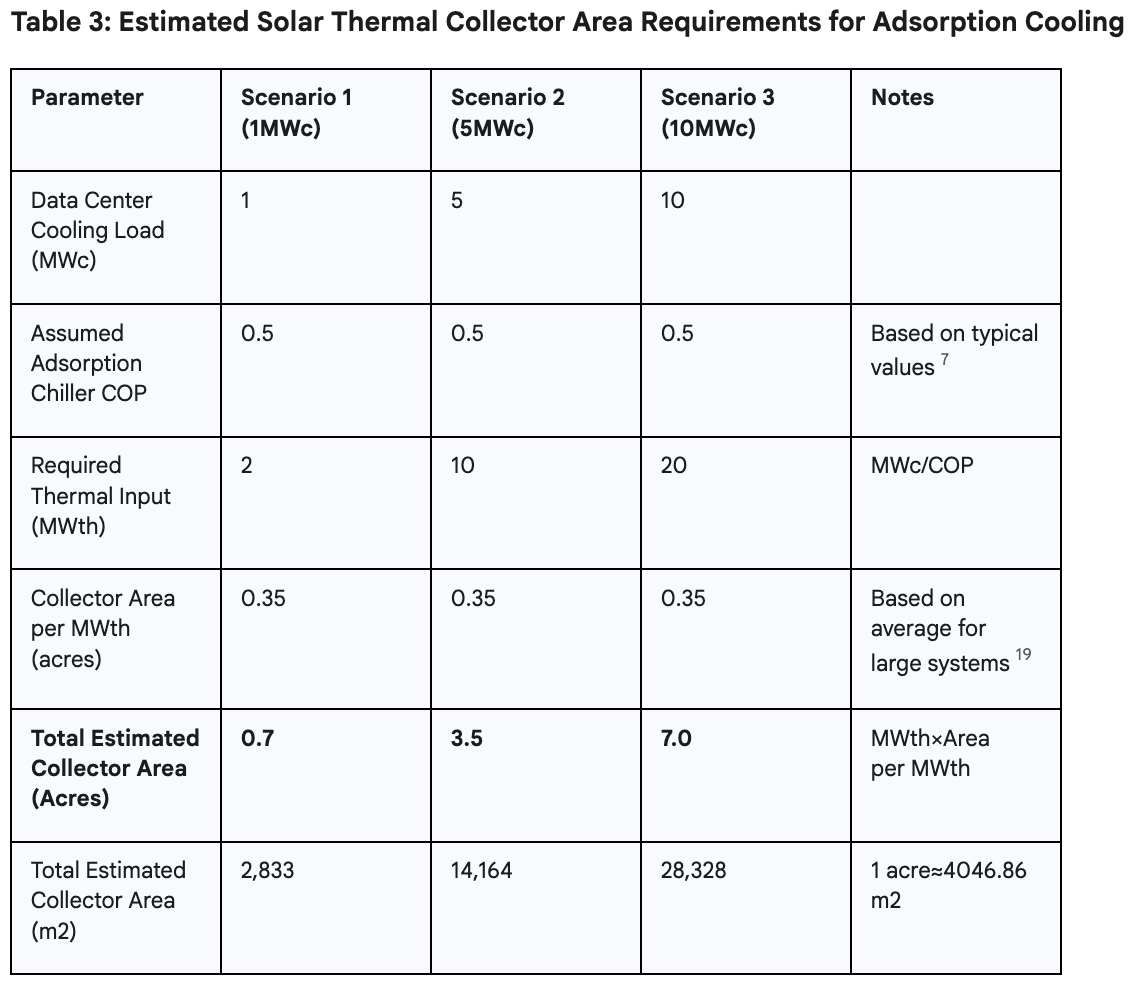
Assumptions: Average solar conditions allowing collectors to meet the required thermal input. Actual area will vary based on specific location, collector type, tracking, and desired solar fraction.
C. Technology Readiness, Scalability, and Risks for Hyperscale Operations
A key concern for hyperscale operators is the current perceived technology readiness and scalability of adsorption chillers for mission-critical, multi-megawatt cooling duties.
- Limited Large-Scale Deployments: While the underlying physics of adsorption cooling is well-established and smaller commercial systems exist, there are few publicly documented large-scale (multi-MW) deployments in 24/7 mission-critical data center environments. Hyperscalers typically demand proven, reliable solutions and are often risk-averse when it comes to core infrastructure like cooling.21
- Scalability: Data center cooling demands are immense. New facilities often require 10 MW or more of cooling capacity from day one, with campuses planned for hundreds of MWs.21 Current commercial adsorption chiller units are often modular, with capacities up to around 1.2 MW (e.g., Bry-Air's 335 TR unit is approximately 1180 kW).7 Scaling to meet a 10 MW, 50 MW, or larger cooling load by deploying numerous smaller modules presents significant engineering, control, and integration challenges. This includes managing complex piping for thermal fluids, ensuring balanced flow, and sophisticated control systems for the entire integrated plant (solar field, thermal storage, multiple chillers). Conventional chiller manufacturers offer single units with capacities exceeding 2.2 MW, providing a benchmark for footprint and capacity that adsorption
systems must strive to match or complement effectively.6
- Operational Risks: For hyperscale operators, reliability and uptime are paramount. Any new cooling technology must demonstrate exceptional reliability under varying IT loads and ambient conditions. The dynamic response of large solar adsorption cooling plants to rapid changes in data center heat load needs to be thoroughly validated. Challenges common to complex cooling systems, such as ensuring even cooling distribution, optimizing energy use, implementing robust real-time monitoring, and managing equipment wear and tear, must be addressed.22
- Grid Impact and Power Density: While adsorption cooling can alleviate the electrical load for chilling, data centers, particularly those supporting AI, still face immense power demands for IT equipment and pose a burden on the electrical grid.3 Adsorption cooling helps with the cooling portion of PUE but doesn't directly address the primary power density on the rack itself.
The "Technology Readiness Level" for hyperscale deployment is not solely about the individual chiller unit but encompasses the entire integrated system—solar farm, thermal storage, chiller plant, and sophisticated controls—operating reliably and efficiently at a massive scale. The lack of extensive public domain case studies for such large, integrated adsorption cooling systems in data centers means that hyperscale operators will likely require comprehensive, multi-year pilot programs, possibly involving co-development partnerships with vendors willing to share the risks and costs of demonstrating such large-scale installations.
D. Vendor Ecosystem and Long-Term Maintenance Profile
The maturity of the vendor ecosystem for adsorption chillers, particularly for large-scale applications, is another consideration.
- Maturing Vendor Landscape: While established manufacturers of smaller adsorption chillers exist 7, the ecosystem for very large, data center-focused systems is still developing compared to the deeply entrenched market for conventional electric chillers. This necessitates careful due diligence by potential adopters regarding vendor stability, manufacturing capacity, long-term technical support, and regional service capabilities.
- Long-Term Maintenance and Material Stability: Adsorption chillers are often marketed as having minimal maintenance due to fewer moving parts (no compressors in the adsorbent beds).7 Bry-Air, for example, claims a life expectancy of over 25 years for their silica gel-based systems.7 However, the long-term performance and durability of adsorbent materials, especially newer ones like MOFs, under continuous cycling in demanding data center environments, need further validation over 10-20 year operational lifecycles. The stability of MOFs in the presence of water refrigerant remains a research area.9 Maintenance of the solar thermal field, pumps, valves, and control systems will also contribute to the overall O&M profile.
Operators need assurance of material stability, consistent performance over the intended lifespan, and availability of spare parts and expert service to de-risk procurement and ensure long-term operational success.
VII. Pathways to Adoption: Pilot Programs and Future Outlook
The transition towards widespread adoption of solar-driven adsorption chillers in data centers will likely be gradual, spearheaded by successful pilot programs and continuous advancements in technology and cost-effectiveness. These efforts are crucial for de-risking the technology and building confidence among data center operators.
A. Designing Effective Pilot Programs: Key Metrics for Evaluation
To move beyond theoretical modeling and laboratory-scale demonstrations, focused pilot programs in real-world data center environments are essential. These programs must be designed to rigorously evaluate the technology's performance, reliability, and economic viability under dynamic operational conditions. Key metrics for evaluation should include:
- Thermal Performance:
- Net cooling output (kW or Tons) under varying seasonal and diurnal IT load profiles.
- Achieved chilled water temperatures and stability.
- Actual average Coefficient of Performance (COP) across different operating conditions (solar availability, ambient temperature, heat load).
- Efficiency and effectiveness of the thermal energy storage system (charging/discharging efficiency, heat loss).
- Dynamic response time of the cooling system to changes in IT heat load.
- Energy Efficiency:
- Overall system Power Usage Effectiveness (PUE) when the adsorption chiller is operational.
- Solar Fraction: The percentage of total cooling energy provided by solar thermal input.
- Parasitic electricity consumption of pumps, fans, and controls (kWh per kWh of cooling).
- Water Efficiency:
- Quantification of any process water consumption (should be negligible for the chiller itself if dry cooling is used for heat rejection).
- Water reclamation performance: Volume and quality of condensate produced per kWh of cooling, particularly in relevant climatic conditions.
- Impact on overall facility Water Usage Effectiveness (WUE).
- Economic Performance:
- Actual installed capital cost (CAPEX) per kW of cooling capacity.
- Operational expenditure (OPEX), including electricity for parasitic loads, maintenance costs (labor and materials), and any consumables.
- Calculated cost per kW of chilled water delivered, compared against existing or conventional systems.
- Operational Viability & Reliability:
- System uptime and availability.
- Mean Time Between Failures (MTBF) and Mean Time To Repair (MTTR) for key components.
- Ease of operation and integration with existing Building Management Systems (BMS) or data center infrastructure management (DCIM) systems.
- Staffing requirements and required skill sets for operation and maintenance.
- Material Durability (Longer-term assessment):
- Monitoring of adsorbent material performance over the pilot duration (e.g., any measurable degradation in adsorption capacity or cycle time, if feasible within the pilot timeframe).
- Assessment of solar thermal collector performance degradation.
Successful pilot programs will not only validate these technical and economic parameters but also help establish clear operational protocols, maintenance schedules, and staff training programs. This holistic approach is critical for building the operational confidence needed for broader adoption, especially within the risk-averse data center industry.
The following table outlines a framework for Key Performance Indicators (KPIs) in such pilot programs:
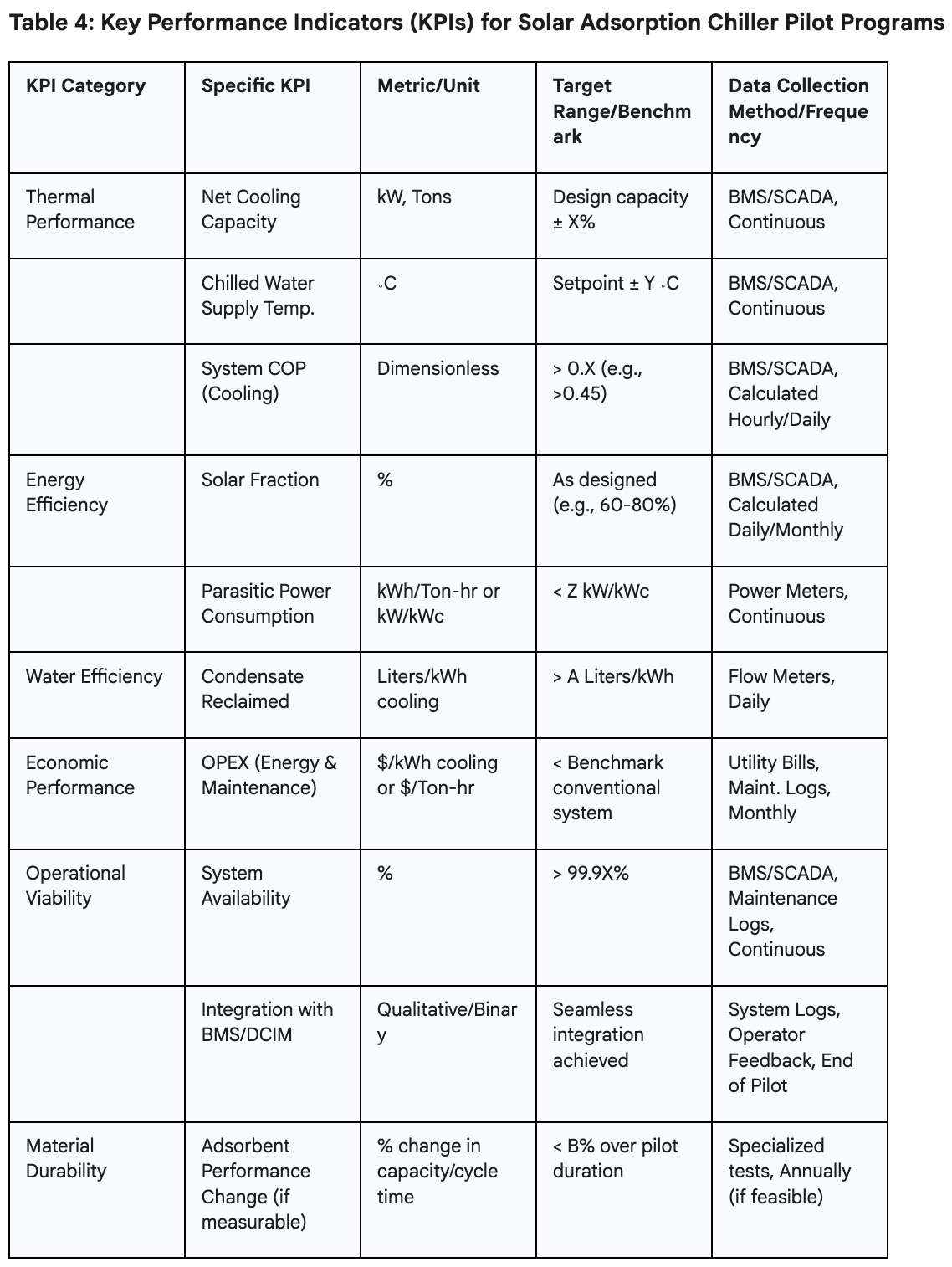
B. Addressing Current Limitations and Areas for Future R&D
Continued research and development are vital to overcome existing limitations and enhance the attractiveness of solar adsorption cooling:
- Material Science: The quest for improved adsorbent materials remains a priority. This includes developing MOFs with higher adsorption capacities for water refrigerant that also exhibit excellent long-term hydrothermal stability.8 Alternatively, optimizing MOFs for use with other environmentally acceptable refrigerants where stability is less of an issue could be pursued. Enhancing the thermal conductivity and mechanical strength of composite adsorbents is also crucial.8
- System Integration and Control: Optimizing the dynamic performance of the entire integrated system—solar collectors, thermal storage, and adsorption chillers—under fluctuating data center loads and variable solar conditions is key. Advanced, smart thermal control algorithms can play a significant role in maximizing efficiency and reliability.
- Scaling and Modularization: There is a need to develop larger, standardized, and modular adsorption chiller units that can be more easily scaled to meet the multi-megawatt cooling demands of modern data centers. The concept of modularity, already prevalent in data center construction and conventional cooling solutions 23, should be applied to adsorption chiller plants to facilitate faster deployment and scalability.
- Cost Reduction: Innovations in materials science, component manufacturing (e.g., adsorbent beds, heat exchangers), and system assembly processes are essential to bring down the initial CAPEX of adsorption cooling systems, making them more competitive with conventional technologies. Future R&D should focus not just on material properties in isolation, but on "design for manufacturability and scalability" of complete adsorption chiller systems. This holistic approach is crucial for reducing CAPEX and meeting the volume demands of the rapidly growing data center industry.
C. The Role of Adsorption Cooling in Achieving Net-Zero Data Centers
Solar-driven adsorption cooling can be a cornerstone technology in the journey towards net-zero and environmentally sustainable data centers.
- By primarily using solar thermal energy, it significantly reduces Scope 2 emissions associated with purchased electricity for cooling.
- When integrated with waste heat recovery from IT equipment or other on-site processes, it improves overall facility energy efficiency and reduces thermal discharge to the environment.
- Its inherent water efficiency directly contributes to water neutrality or water positivity goals, a critical factor in water-stressed regions.
- The technology aligns with the broader trends of renewable energy integration, circular economy principles (waste heat as a resource), and resilient infrastructure development.
As the data center industry faces increasing scrutiny over its environmental footprint, solutions like solar adsorption cooling offer a tangible pathway to demonstrate commitment to sustainability, reduce operational risks associated with resource volatility, and potentially gain a competitive edge.
VIII. Conclusion and Strategic Recommendations
Solar-driven adsorption chillers present a technologically viable and environmentally compelling alternative to conventional data center cooling methods. By leveraging solar thermal energy or waste heat, these systems offer the potential to significantly reduce electricity consumption, operational costs, water usage, and carbon emissions associated with data center cooling—a critical component of a facility's energy footprint. The synergy with direct-to-chip liquid cooling, which provides high-grade waste heat suitable for driving adsorption cycles, further enhances their attractiveness in modern, high-density data center designs.
The benefits are substantial: drastically lower electrical OPEX due to "free" thermal energy input, minimal water consumption in the core chilling process, reduced reliance on synthetic refrigerants, and the ability to contribute to peak load shaving when combined with thermal storage. These advantages position adsorption cooling as a strategic response to multiple converging pressures: volatile energy prices, increasing water scarcity, stringent environmental regulations, and the growing demand for sustainable data processing.
However, significant challenges must be addressed to unlock this potential, particularly for widespread adoption in hyperscale facilities. These include higher initial capital expenditures compared to traditional systems, the substantial land footprint required for solar thermal collector arrays, and the current state of technology readiness and vendor ecosystem maturity for very large-scale, mission-critical deployments. The long-term durability of advanced adsorbent materials under demanding operational conditions also requires further validation. The adoption pathway will likely be phased, commencing with niche applications or new builds in geographically favorable regions (high solar irradiance, water stress), and gradually expanding as the technology matures, costs decrease, and operational confidence is established through successful deployments. It is unlikely to be an immediate, wholesale replacement for conventional cooling.
To accelerate the adoption and realize the benefits of solar-driven adsorption cooling, the following strategic recommendations are proposed:
- For Data Center Operators in High-DNI / Water-Stressed Regions:
- Prioritize comprehensive feasibility studies for integrating solar adsorption cooling in new data center builds or major expansions.
- Initiate and invest in well-instrumented pilot programs to validate performance, reliability, and economic benefits under local climatic and operational conditions. These pilots are crucial for de-risking the technology and building internal expertise.
- For Hyperscale Operators:
- Engage proactively with adsorption chiller manufacturers and research institutions to co-develop and pilot large-scale (multi-MW) systems tailored to hyperscale requirements.
- Focus pilot programs on demonstrating sustained reliability, scalability of modular designs, dynamic load response, and seamless integration with existing data center management and control systems.
- For R&D Institutions and Manufacturers:
- Intensify research efforts on developing next-generation adsorbent materials with higher capacity, improved hydrothermal stability (especially for water refrigerant), and lower manufacturing costs.
- Focus on engineering advancements to reduce the overall system CAPEX, improve COP, and develop standardized, modular chiller designs that facilitate scalability and rapid deployment.
- Collaborate on establishing industry standards and best practices for the design, installation, and operation of large-scale solar adsorption cooling plants.
- For Policymakers and Regulatory Bodies:
- Consider implementing financial incentives, such as tax credits or grants, to encourage the adoption of low-emission, water-efficient cooling technologies like solar adsorption chillers in data centers.
- Support R&D initiatives and demonstration projects that advance the technology and reduce its cost.
- Streamline permitting processes for renewable energy installations associated with data centers.
The urgency to find sustainable cooling solutions is driven not only by environmental responsibility but also by economic and competitive factors. Data centers that successfully integrate innovative, resource-efficient technologies like solar adsorption cooling will be better positioned to manage operational costs, meet evolving regulatory requirements, satisfy customer demands for sustainable services, and secure their social license to operate in an increasingly resource-constrained world. The opportunity to transform data center cooling is significant—passively powerful in its use of ambient energy, elegantly simple in its core principles, and increasingly urgent in its necessity. Proactive engagement, targeted investment, and collaborative innovation are key to unlocking this promise.
Work Cited
Datacenter Anatomy Part 2 – Cooling Systems - SemiAnalysis, accessed May 7, 2025, https://semianalysis.com/2025/02/13/datacenter-anatomy-part-2-cooling-systems/
kth.diva-portal.org, accessed May 7, 2025, https://kth.diva-portal.org/smash/get/diva2:1882351/FULLTEXT01.pdf
Data Centers AI & ML Trilemma - GE Vernova, accessed May 7, 2025, https://www.gevernova.com/content/dam/gepower-new/global/en_US/downloads/gas-new-site/industries/GEA35355-Data-Centers-AI-ML-Trilemma-WP.pdf
Reducing Data Center Peak Cooling Demand and Energy Costs With Underground Thermal Energy Storage | News | NREL, accessed May 7, 2025, https://www.nrel.gov/news/program/2025/reducing-data-center-peak-cooling-demand-and-energy-costs-with-underground-thermal-energy-storage.html
A Viability Study of Modular Datacentre Cooling System using Absorption Chiller Integrated with Solar Thermal Collectors - UPCommons, accessed May 7, 2025, https://upcommons.upc.edu/bitstream/handle/2117/425595/SupriyaSomanThesis.pdf?sequence=2&isAllowed=y
Cooling the future: how high-capacity chillers are shaping tomorrow's data centers and industry - Vertiv, accessed May 7, 2025, https://www.vertiv.com/en-us/about/news-and-insights/articles/blog-posts/cooling-the-future-how-high-capacity-chillers-are-shaping-tomorrows-data-centers-and-industry/
Desiccant Adsorption Chillers - Bry-Air, accessed May 7, 2025, https://www.bryair.com/products/desiccant-adsorption-chillers/
rjes.iq, accessed May 7, 2025, https://rjes.iq/index.php/rjes/article/download/145/136/1271
In Silico Screening of Metal–Organic Frameworks for Adsorption ..., accessed May 7, 2025, https://pubs.acs.org/doi/10.1021/acsami.8b09343
Design and performance of a solar-powered heating and cooling ..., accessed May 7, 2025, https://www.researchgate.net/publication/238175114_Design_and_performance_of_a_solar-powered_heating_and_cooling_system_using_silica_gelwater_adsorption_chiller
Absorption chiller costs and economics? - Thunder Said Energy, accessed May 7, 2025, https://thundersaidenergy.com/downloads/absorption-chillers-the-economics/
Data Center Waste Heat Reuse: An Investment Analysis | J. Eng. Sustain. Bldgs. Cities, accessed May 7, 2025, https://asmedigitalcollection.asme.org/sustainablebuildings/article/6/2/021002/1210426/Data-Center-Waste-Heat-Reuse-An-Investment
Total Cost of Ownership Prediction in Chilled Water Plants: Contributing Factors and Role of Artificial Intelligence - MDPI, accessed May 7, 2025, https://www.mdpi.com/2076-3417/15/3/1618
Economic costs of data-centers? - Thunder Said Energy, accessed May 7, 2025, https://thundersaidenergy.com/downloads/data-centers-the-economics/
The value of air conditioning - Frontiers, accessed May 7, 2025, https://www.frontiersin.org/journals/energy-efficiency/articles/10.3389/fenef.2024.1471628/full
THEORETICAL PERFORMANCE OPTIMIZATION OF SOLAR ABSORPTION CHILLER COUPLED TO UNDERGROUND COOLING TOWER - IYTE GCRIS Database, accessed May 7, 2025, https://gcris.iyte.edu.tr/bitstream/11147/7188/1/T001868.pdf
Using the sun to power data centers - Consulting - Specifying Engineer -, accessed May 7, 2025, https://www.csemag.com/using-the-sun-to-power-data-centers/
How Much Solar Power Would It Take to Run a Hyperscale Data Center - Simple Thread %, accessed May 7, 2025, https://www.simplethread.com/fun-friday-how-much-solar-power-would-it-actually-take-to-power-a-hyperscale-data-center/
SOLAR HEAT WORLD WIDE - IEA SHC, accessed May 7, 2025, https://www.iea-shc.org/data/sites/1/publications/Solar-Heat-Worldwide-2022.pdf
Understanding the transformative potential of solar thermal technology for urban sustainability - Frontiers, accessed May 7, 2025, https://www.frontiersin.org/journals/sustainable-cities/articles/10.3389/frsc.2025.1583316/full
North America Data Center Trends H2 2024 | CBRE, accessed May 7, 2025, https://www.cbre.com/insights/reports/north-america-data-center-trends-h2-2024
Top 5 Challenges of Cooling Modern Data Centers and How Flow Meters Solve Them, accessed May 7, 2025, https://www.onicon.com/education-series/top-5-challenges-of-cooling-modern-data-centers-and-how-flow-meters-solve-them
Modular Data Centers Solutions - BASX Solutions, accessed May 7, 2025, https://basxsolutions.com/modular-data-centers-solutions/
Chillers and Generators for Data Centers :: Tecogen, Inc. (TGEN), accessed May 7, 2025, https://www.tecogen.com/markets/datacenter-generators-chillers